RSS-Feed abonnieren

DOI: 10.1055/s-0036-1590969
Diastereoselective [Cu(MeCN)4BF4/BF3·Et2O]-Catalyzed Cyclopropenation of Alkynes: Asymmetric Synthesis of β-Amino-α-cyclopropenyl Phosphonates
We thank the Committee of Science and Technology of Tianjin (15JCYBJC20700), the Tianjin Rural Work Committee (201604020) and State Key Laboratory of Elemento-Organic Chemistry in Nankai University for financial support.
Publikationsverlauf
Received: 31. Mai 2017
Accepted after revision: 06. Juli 2017
Publikationsdatum:
03. August 2017 (online)
Abstract
The diastereospecific formation of β-amino-α-cyclopropenyl phosphonates has been achieved in moderate yields from the cyclopropenation of 1-alkynes with dialkyl α-diazophosphonates. The reaction was performed by using a combined catalyst consisting of Cu(MeCN)4BF4 and BF3·Et2O as additive in dichloromethane at 40 °C. A possible mechanism for the reaction has been proposed to explain the origin of the activation and the asymmetric induction. This method provides a versatile approach to β-amino-α-cyclopropenylphosphonates containing a quaternary stereogenic center with good efficiency and diastereoselectivity.
Key words
cyclopropenation - α-diazophosphonates - asymmetric synthesis - cyclopropenylphosphonates - Cu(MeCN)4BF4/BF3·Et2OCyclopropenes are a unique class of the smallest ring compound with a double bond, and the combination of high strain and unsaturation renders cyclopropenes as versatile synthons in a wide variety of organic synthesis.[1] To enhance the synthetic potential of cyclopropenes further, the development of expeditious methods for their synthesis is highly desirable.[2] One of the most efficient catalytic asymmetric methods for their preparation involves the reaction of alkynes with diazo compounds using chiral copper(I),[3] iridium(II),[4] cobalt(II),[5] or rhodium(II)[6] catalysts. Diazo compounds are commonly used in formation of metallocarbenoids, which can subsequently undergo diverse chemical transformations.[7] In 1992, Doyle, Müller and co-workers reported the first cyclopropenation reactions of diazoacetates with terminal alkynes using [Rh2(5S-mepy)4] as a chiral catalyst.[8] Corey and co-workers also reported a significantly improved enantioselective cyclopropenation of ethyl diazoacetate with 1-alkynes using a new rhodium complex, Rh2(OAc)(DPTI)3, as a catalyst.[9] Although high levels of enantioselectivity (up to 99% ee) in cyclopropenations of terminal alkynes with aryldiazoacetates under catalysis by [Rh2(S-dosp)4] have been reported by Davies and co-workers,[10] stereoselective syntheses of the corresponding cyclopropenylphosphonates using such a strategy remain scarce.[11]
α-Diazophosphonates are phosphorus analogues of α-diazoacetates, and therefore could undergo a range of chemical transformations and the synthesis of varieties of functionalized phosphorous compounds.[12] In 2013, Charette and co-workers reported the first catalytic asymmetric synthesis of bis-acceptor cyclopropylphosphonates through the reaction of α-cyano diazophosphonates with allenes catalyzed by Rh2(S-IBAZ)4 under mild reaction conditions.[13] Cyclopropylphosphonates demonstrate a range of biological and biochemical properties.[14] By analogy with cyclopropylphosphonates,[15] the development of efficient methods for the preparation of cyclopropenephosphonates is attracting increasing attention. In particular, the presence of a quaternary stereocenter in a highly substituted cyclopropenephosphonate can lead to interaction with certain proteases and a resistance to proteolytic degradation.[14]
Recently, we reported novel α-diazophosphonyl compounds prepared from natural amino acids that be used to could perform combined C–H functionalization and O–H insertion to form tertiary β-alkoxy-substituted β-aminophosphonates (Scheme [1], Eq. 1).[16] As a natural extension of the combined C–H functionalization/O–H insertion reaction, we have developed a regioselective boron trifluoride-catalyzed C–H functionalization/S–H insertion reaction for the synthesis of N,S-acetals containing quaternary centers (Scheme [1], Eq. 2).[17] In a continuation of our interest in the chemistry of aminophosphorus derivatives,[18] we herein report the first example of the conversion of dialkyl α-diazophosphonates into cyclopropenylphosphonate derivatives catalyzed by tetrakis (acetonitrile)copper(I) tetrafluoroborate/boron trifluoride [Cu(MeCN)4BF4/BF3·Et2O] in a highly diastereoselective manner (Scheme [1], Eq. 3).


We began our investigations by studying the reaction of diethyl α-diazophosphonate 1a [16] with phenylacetylene 2a in the presence of catalyst in dichloromethane at 25 °C (Table [1]). The results revealed that transition-metal catalysts such as Rh2(OAc)4 and Hg(OTf)2 did not decompose 1a in the presence of 2a (entries 1 and 2). The other catalysts tested (e.g., CuOTf, AgOTf, and Cu(MeCN)4PF6) could be used to promote the reaction, resulting in low yields of product with moderate diastereoselectivities (entries 3–5). To our delight Cu(MeCN)4BF4 catalyzed the decomposition of 1a, and diethyl(2-(phenyl-cycloprop-2-enyl)-2-(1,3-dioxoisoindolin-2-yl)ethyl)phosphonate (3a) was obtained with good diastereoselectivity (dr = 12:1) in 29% yield (entry 6). In addition, a 1,2-hydrogen migration by-product (Z)-diethyl(2-(1,3-dioxoisoindolin-2-yl)prop-1-en-1-yl) phosphonate (4a) was obtained in 9% yield. The molecular structure of 3a was determined based on 1H, 31P, 13C NMR, and 2D NMR spectroscopy and mass spectrometry.[19] This result encouraged us to choose Cu(MeCN)4BF4 as the catalyst for further optimization of the reaction conditions. To improve the reactivity and diastereoselectivity, the effects of different additives and solvents were investigated.
Initially, additives were evaluated to study the diastereoselectivity of this transformation. As shown in Table [1], both the diastereoselectivity and the ratio of the two products 3a and 4a was affected dramatically by the additive. In the presence of Cu(MeCN)4BF4 as the catalyst (5 mol%) and BF3·Et2O (20 mol%) as the additive in dichloromethane, the reaction proceeded with an increased combined yield and good diastereoselectivity (dr = 12:1) (entry 7). Furthermore, we found that high temperatures favored this reaction. When the reaction was carried out at 40 °C, the desired product 3a could be obtained in 66% yield (entry 8). After screening BF3·Et2O, it was found that acetone, CH3CN, DMF, and iodine did not give superior results in terms of either reactivity or stereoselectivity (entries 9–12).
a Unless otherwise specified, all reactions were carried out using α-diazophosphonate 1a (0.28 mmol, 1 equiv) and phenylacetylene 2a (1.40 mmol, 5 equiv) in the specified solvent (6 mL) with 5 mol% catalyst and 20 mol% additive at 25 °C for 3.5 h (addition over 1.5 h, then a further 2 h).
b The product ratio was determined by 31P NMR spectroscopic analysis of the crude product.
c Overall yield of the mixture of 3a and 4a after silica gel chromatograph.
d Yield of isolated product.
e Diastereomeric ratio (dr) based on 31P NMR spectroscopic analysis of the isolated product 3a.
f Reaction temperature 40 °C.
g DME = dimethoxyethane.
h 2 mol% Cu(MeCN)4BF4 and 10 mol% BF3·Et2O were used.
i 10 mol% Cu(MeCN)4BF4 and 30 mol% BF3·Et2O were used.
With the optimum combination of Cu(MeCN)4BF4 catalyst and BF3·Et2O additive, we next carried out the reaction in different solvents to determine the best solvent for this reaction. Toluene, THF, DME, and 1,2-dichloroethane all provided lower yields of the desired products 3a and 4a with moderate diastereoselectivities (Table [1], entries 13–16). This screening therefore identified CH2Cl2 as the optimal solvent for this reaction. Furthermore, a decrease in the catalyst loading to 2 mol% Cu(MeCN)4BF4 and 10 mol% BF3·Et2O led to a decrease in both yield and diastereoselectivity (entry 17). A similar result was obtained when the reaction was performed in CH2Cl2 with increased catalyst loading to 10 mol% Cu(MeCN)4BF4 and 30 mol% BF3·Et2O (entry 18). Thus, the optimal reaction conditions for this transformation were determined to be 0.28 mmol α-diazo phosphonate 1a, 5.0 equivalents of phenylacetylene 2a, 5 mol% Cu(MeCN)4BF4 and 20 mol% BF3·Et2O as co-catalyst in 6 mL CH2Cl2 as solvent at 40 °C.
Under the optimal reaction conditions, the substrate scope of this reaction was investigated (Table [2]). The impact of different groups on the β-position of dialkyl α-diazophosphonates 1 was evaluated. α-Diazophosphonates 1a–e with different substituents in the β-position, such as methyl, benzyl, isobutyl, ethyl, and 4-acetoxybenzyl groups, afforded moderate yields of the β-amino-α-cyclopropenylphosphonates 3a–e and the 1,2-hydride migration products 4a–e (entries 1–5). It is worth noting that diethyl α-diazophosphonate 1f, derived from methionine, did not undergo reaction to give the desired products, probably due to the fact that the sulfur atom coordinates with the copper(I) and hinders the reaction (entry 6). Instead, the starting material 1f decomposed under the reaction conditions. In addition to phenylacetylene, 3-methylphenyl acetylene and 4-methylphenylacetylene were also examined in the cyclopropenation reaction with carbenoids derived from dialkyl α-diazophosphonates 1b and 1g. Electron-rich phenylacetylene derivatives, substituted either at the meta-, or para-position, all provided good yields and diastereoselectivities of the corresponding β-amino-α-cyclopropenylphosphonates (entries 7 and 8). Chiral cyclopropene derivatives 3i and 3j from reactions of aromatic alkynes containing both electron-withdrawing and -donating groups at the para-position could also be obtained in poor yields but with good diastereoselectivities (entries 9 and 10). The cyclopropenation could also be conducted on the internal alkyne prop-1-ynyl-benzene 2f with excellent diastereoselectivity (>30:1), albeit in diminished yield (11%), presumably due to the steric effect (entry 11).
To assess the effects of the substrate on product selectivity, we set out to study the reactions of a series of dialkyl α-diazophosphonates 1h–l under Cu(MeCN)4BF4/BF3·Et2O catalysis. When the steric bulk of the R2 group was increased from methyl to isopropyl or n-butyl, similar yields were obtained. These results showed that the size of the R2 group on the α-diazophosphonates 1 has almost no influence on reactivity in this cyclopropenation reaction (Table [2], entries 12–16). The method was successful for alkynes that are conjugated to an arene. However, cyclopropene products were not obtained when diethyl α-diazophosphonate 1a was reacted with (triisopropyl)silylacetylene or 1-hexyne.
a Reaction conditions: α-diazophosphonate 1 (0.28 mmol) and 2 (1.40 mmol, 5 equiv) in CH2Cl2 (6 mL) at 40 °C in the presence of 5 mol% Cu(MeCN)4BF4 and 20 mol% BF3·Et2O for 3.5 h (addition over 1.5 h, then a further 2 h).
b The product ratio was based on 31P NMR spectroscopic analysis of the crude product.
c Overall yield of the mixture of 3 and 4 after silica gel chromatography.
d Yield of isolated product.
e Diastereomeric ratio (dr) based on 31P NMR spectroscopic analysis of the isolated product 3a.
A stepwise mechanism for the formation of the cyclopropenylphosphonates is outlined in Scheme [2]. Firstly, α-diazophosphonate 1 releases N2 under the influence of the Cu catalyst on heating, affording Cu-carbenoid A.[20] The role of the strong Lewis acids (BF3·Et2O) could be to coordinate to the copper to give metallocarbenoid B in order to make the α-diazo position more electron deficient. Carbenoid B is then trapped by the triple bond of ethynylbenzene to give vinyl cation C. Subsequent cleavage of the C–Cu bond of intermediate C with delivery of the electrons to the vinyl cation leads to cyclopropenyl phosphonate 3 with simultaneous regeneration of the Cu(I) catalyst and BF3·Et2O. Intermediate B may also be transformed into by-product 4 through loss of Cu(MeCN)4BF4 and BF3·Et2O before the attack of phenylacetylene 2a.
The diastereoselectivity observed in generating the cyclopropenyl phosphonates implies that conformational factors may play a role in the cyclopropenation process. In fact, the stereoselectivity can be attributed to steric hindrance between the phosphonate group and the R1 group, with attack occurring on the back face of the carbenoid preferentially. In forming intermediate C, the terminal alkyne carbon is involved in C–C bond formation with the carbenoid carbon, while positive charge build-up occurs at the internal sp-carbon. Furthermore, the second C–C bond forms with inversion of the carbenoid center. This model is partly consistent with the observed sense of asymmetric induction, but there are a few anomalies. For instance, isopropyl phosphonate 1j leads to lower dr than methyl phosphonate 1i (Table [2], entries 13 and 14).
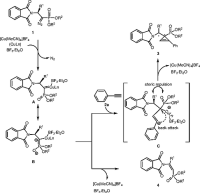

In conclusion, we have developed a Cu(MeCN)4BF4/BF3·Et2O-catalyzed cyclopropenation of 1-alkynes with dialkyl α-diazophosphonates to synthesize β-amino-α-cyclopropenyl phosphonates in moderate yields with good to excellent diastereoselectivities. The observed sense of asymmetric induction is rationalized by invoking steric hindrance between the phosphonate group and the R1 group of the α-diazophosphonate. The present catalytic protocol provides attractive and easy access to β-amino-α-cyclopropenyl phosphonates containing a quaternary stereogenic center. Further studies to explore the enantioselective synthesis of cyclopropenyl phosphonates are in progress in our laboratory.
All reactions and manipulations were performed using standard Schlenk techniques. Solvents were dried and distilled prior to use according to standard methods. Unless otherwise indicated, all materials were obtained from commercial sources, and used as purchased without dehydration. Flash column chromatography was performed on silica gel (particle size 10–40 μm, Ocean Chemical Factory of Qingdao, China). Nitrogen gas (99.999%) was purchased from Boc Gas Inc. 1H NMR, 13C NMR, and 31P NMR spectra were recorded in CDCl3 with Bruker 400 MHz spectrometers, TMS served as internal standard (δ = 0 ppm) for 1H NMR and 13C NMR, H3PO4 served as internal standard (δ = 0 ppm) for 31P NMR. Mass spectra were recorded with a LCQ advantage spectrometer with ESI resource. HRMS were recorded with a PEXII and ZAB-HS spectrometer.
Preparation of 3 and 4; General Procedure
In an oven-dried Schlenk tube, [Cu(MeCN)4]BF4 (0.014 mmol) and BF3·Et2O (0.056 mmol) were dissolved in freshly distilled CH2Cl2 (4 mL) under nitrogen, the requisite phenylacetylene 2 (1.4 mmol) was added and the solution was stirred for 30 min at 25 °C. α-Diazophosphonate 1 (0.28 mmol) dissolved in CH2Cl2 (2 mL) was then added to the reaction mixture dropwise over a period of 1.5 h by using a syringe pump. When the addition was complete, the reaction mixture was stirred for a further 2 h at 40 °C. The solvent was then removed under reduced pressure and the crude residue was purified by silica gel chromatography, eluting with CH2Cl2/EtOAc (15:1) to afford the corresponding products 3 and 4.
Diethyl(1-(phenylcycloprop-2-enyl)-2-(1,3-dioxoisoindolin-2-yl)ethyl)phosphonate (3a)
Yield: 0.085 g (66%); pale-yellow oil.
1H NMR (400 MHz, CDCl3): δ = 7.61 (dd, J = 5.2, 3.2 Hz, 2 H), 7.56 (dd, J = 5.3, 3.1 Hz, 2 H), 7.49 (d, J = 7.1 Hz, 2 H), 7.16–7.25 (m, 3 H), 7.01 (d, J = 4.1 Hz, 1 H), 5.17–5.27 (m, 1 H, CH), 4.11 (dd, J = 12.8, 5.7 Hz, 4 H), 1.70 (d, J = 7.2 Hz, 3 H), 1.30 (td, J = 7.0, 4.2 Hz, 6 H).
13C NMR (101 MHz, CDCl3): δ = 168.35, 133.57, 131.51, 129.77, 129.59, 128.30, 125.48, 122.71, 116.00, 97.61, 62.02, 61.88, 47.44 (d, J = 36.5 Hz), 30.15, 28.17, 16.16.
31P NMR (162 MHz, CDCl3): δ = 26.86.
HRMS (ESI): m/z [M + H]+ calcd for C23H24NO5P: 426.1426; found: 426.1470.
Diethyl(1-(phenylcycloprop-2-enyl)-2-(1,3-dioxoisoindolin-2-yl)-2-phenylethyl)phosphonate (3b)
Yield: 0.086 g (58%); pale-yellow oil.
1H NMR (400 MHz, CDCl3): δ = 7.49 (d, J = 6.5 Hz, 5 H), 7.11–7.28 (m, 7 H), 7.09 (d, J = 4.0 Hz, 2 H), 5.43 (d, J = 11.0 Hz, 1 H), 4.17 (dd, J = 15.5, 7.7 Hz, 4 H), 3.06–3.83 (m, 2 H), 3.51 (dd, J = 14.2, 4.0 Hz), 1.32–1.38 (m, 6 H).
13C NMR (101 MHz, CDCl3): δ = 137.33, 133.40, 132.40, 128.71, 128.58, 127.66, 127.30, 125.21, 124.36, 122.66, 121.58, 114.55, 96.66, 61.04, 52.60 (d, J = 45.4 Hz), 33.87, 28.68, 15.44.
31P NMR (162 MHz, CDCl3): δ = 26.57.
HRMS (ESI): m/z [M + H]+ calcd for C29H28NO5P: 502.1739; found: 502.1783.
Diethyl(1-(phenylcycloprop-2-enyl)-2-(1,3-dioxoisoindolin-2-yl)-3-methylbutyl)phosphonate (3c)
Yield: 0.078 g (56%); pale-yellow oil.
1H NMR (400 MHz, CDCl3): δ = 7.50–7.60 (m, 4 H), 7.43 (d, J = 6.7 Hz, 2 H), 7.08–7.23 (m, 3 H), 6.97 (d, J = 4.2 Hz, 1 H), 5.17 (d, J = 12.2 Hz, 1 H), 4.31 (t, J = 6.7 Hz, 1 H), 4.04–4.15 (m, 4 H), 2.50 (t, J = 13.3 Hz, 1 H), 2.34 (t, J = 7.4 Hz, 1 H), 1.73 (dd, J = 13.2, 9.3 Hz, 3 H), 1.44 (dd, J = 15.4, 7.6 Hz, 3 H), 0.88–0.93 (m, 6 H).
13C NMR (101 MHz, CDCl3): δ = 168.65, 133.52, 129.68, 129.47, 128.85, 128.26, 125.45, 122.68, 115.62, 97.48, 65.58, 61.97, 50.16 (d, J = 36.2 Hz), 37.45, 29.71, 25.44, 23.59, 22.70, 21.11, 16.48, 14.13, 13.74.
31P NMR (162 MHz, CDCl3): δ = 26.80.
HRMS (ESI): m/z [M + H]+ calcd for C26H30NO5P: 468.1895; found: 468.1924.
Diethyl(1-(phenylcycloprop-2-enyl)-2-(1,3-dioxoisoindolin-2-yl)propyl)phosphonate (3d)
Yield: 0.079 g (60%); pale-yellow oil.
1H NMR (400 MHz, CDCl3): δ = 7.54–7.64 (m, 4 H), 7.47 (d, J = 6.7 Hz, 2 H), 7.15–7.24 (m, 3 H), 6.99 (d, J = 4.2 Hz, 1 H), 4.92–5.03 (m, 1 H), 4.10 (ddd, J = 14.5, 11.7, 7.1 Hz, 4 H), 2.35–2.42 (m, 1 H), 2.06–2.14 (m, 1 H), 1.34–1.49 (m, 3 H), 0.87 (dd, J = 15.3, 7.9 Hz, 6 H).
13C NMR (101 MHz, CDCl3): δ = 168.66, 133.56, 131.39, 129.72, 129.52, 128.29, 125.55, 122.71, 115.64, 97.64, 61.97, 53.99 (d, J = 36.1 Hz), 22.71, 22.11, 14.14, 11.20.
31P NMR (162 MHz, CDCl3): δ = 26.94.
HRMS (ESI): m/z [M + H]+ calcd for C24H26NO5P: 440.1582; found: 440.1619.
(2-(Diethoxyphosphoryl)-1-(1,3-dioxoisoindolin-2-yl)-2-(phenylcycloprop-2-enyl)ethyl)phenyl Acetate (3e)
Yield: 0.087 g (54%); pale-yellow oil.
1H NMR (400 MHz, CDCl3): δ = 7.42–7.49 (m, 5 H), 7.14–7.21 (m, 4 H), 6.96–7.11 (m, 2 H), 6.82–6.86 (m, 2 H), 5.35–5.42 (m, 1 H), 4.13 (dt, J = 7.1, 4.7 Hz, 4 H), 3.07–3.94 (m, 1 H), 3.69 (dd, J = 14.2, 12.6 Hz, 1 H), 3.47 (dd, J = 14.4, 4.2 Hz, 1 H), 2.20 (s, 3 H), 1.32 (dd, J = 7.0, 3.5 Hz, 6 H).
13C NMR (101 MHz, CDCl3): δ = 169.37, 149.05, 135.95, 133.67, 133.50, 130.69, 129.75, 129.64, 128.95, 128.33, 125.27, 122.68, 121.36, 115.53, 97.54, 62.07, 53.44 (d, J = 36.9 Hz), 34.38, 29.72, 21.10, 16.54.
31P NMR (162 MHz, CDCl3): δ = 26.52.
HRMS (ESI): m/z [M + H]+ calcd for C31H30NO7P: 560.1793; found: 560.1838.
Diethyl(1-(2-m-tolylcycloprop-2-enyl)-2-(1,3-dioxoisoindolin-2-yl)-2-phenylethyl)phosphonate (3g)
Yield: 0.095 g (62%); pale-yellow oil.
1H NMR (400 MHz, CDCl3): δ = 7.47 (s, 4 H), 7.24 (s, 2 H), 7.11–7.20 (m, 4 H), 7.02–7.09 (m, 3 H), 6.94 (d, J = 7.7 Hz, 1 H), 5.35–5.45 (m, 1 H), 4.09–4.19 (m, 4 H), 3.61–3.70 (m, 1 H), 3.47 (dd, J = 14.2, 4.2 Hz, 1 H), 2.18 (s, 3 H), 1.33 (td, J = 7.0, 3.5 Hz, 6 H).
13C NMR (101 MHz, CDCl3): δ = 138.36, 137.91, 133.38, 130.41, 130.35, 128.70, 128.32, 128.26, 126.90, 126.23, 125.20, 123.70, 122.55, 115.48, 97.31, 62.03, 53.54 (d, J = 37.3 Hz), 34.96, 29.71, 21.05, 16.52.
31P NMR (162 MHz, CDCl3): δ = 26.79.
HRMS (ESI): m/z [M + H]+ calcd for C30H30NO5P: 516.1895; found: 516.1912.
Dibutyl(1-(2-p-tolylcycloprop-2-enyl)-2-(1,3-dioxoisoindolin-2-yl)-2-phenylethyl)phosphonate (3h)
Yield: 0.108 g (64%); pale-yellow oil.
1H NMR (400 MHz, CDCl3): δ = 7.49 (s, 4 H), 7.13–7.27 (m, 5 H), 7.07 (dt, J = 10.2, 5.8 Hz, 3 H), 6.96 (d, J = 7.4 Hz, 1 H), 5.43 (d, J = 12.3 Hz, 1 H), 4.00–4.18 (m, 4 H), 3.64–3.74 (m, 1 H), 3.51 (dd, J = 14.3, 4.1 Hz, 1 H), 2.37 (t, J = 7.4 Hz, 1 H), 2.21 (s, 3 H), 1.35–1.52 (m, 8 H), 0.94 (t, J = 7.2 Hz, 6 H).
13C NMR (101 MHz, CDCl3): δ = 171.20, 138.37, 137.87, 133.36, 130.93, 130.36, 128.85, 128.68, 128.31, 128.21, 126.90, 126.21, 122.54, 97.22, 65.80, 60.42, 53.53 (d, J = 37.2 Hz), 34.97, 32.66, 31.93, 29.70, 22.70, 21.07, 18.79, 14.20, 13.65.
31P NMR (162 MHz, CDCl3): δ = 26.74.
HRMS (ESI): m/z [M + H]+ calcd for C34H38NO5P: 572.2521; found: 572.2569.
Diethyl(1-(4-fluorophenylcycloprop-2-enyl)-2-(1,3-dioxoisoindolin-2-yl)ethyl)phosphonate (3i)
Yield: 0.019 g (19%); pale-yellow oil.
1H NMR (400 MHz, CDCl3): δ = 7.50–7.56 (m, 4 H), 7.41 (dd, J , 7.41 (dd Hz, 2 H), 6.82–6.89 (m, 3 H), 5.11 (d, J = 7.1 Hz, 1 H), 3.97–4.05 (m, 4 H), 1.60 (d, J , 1.6 Hz, 3 H), 1.18–1.24 (m, 6 H).
13C NMR (101 MHz, CDCl3): δ = 168.30, 133.67, 131.86, 131.78, 131.51, 122.74, 115.57 (d, J 168.30 Hz), 97.17, 61.96 (dd, J = 18.1, 6.9 Hz), 47.39 (d, J z), 47 Hz), 30.20, 28.23, 16.42.
31P NMR (162 MHz, CDCl3): δ = 26.56.
HRMS (ESI): m/z [M + H]+ calcd for C23H24FNO5P: 444.1371; found: 444.1378.
Diethyl(1-(4-methoxyphenylcycloprop-2-enyl)-2-(1,3-dioxoisoindolin-2-yl)-2-phenylethyl)phosphonate (3j)
Yield: 0.030 g (25%); pale-yellow oil.
1H NMR (400 MHz, CDCl3): δ = 7.40–7.48 (m, 5 H), 7.13–7.19 (m, 4 H), 7.09–7.10 (m, 1 H),7.05 (t, J (t, Hz, 1 H), 6.89 (d, J (d, Hz, 1 H), 6.71–6.79 (m, 2 H), 5.38 (d, J (d, ( Hz, 1 H), 4.12 (dd, J (dd, (400 M Hz, 4 H), 3.72 (s, 3 H), 3.62–3.69 (m, 1 H), 3.44–3.53 (m, 1 H), 1.29–1.34 (m, 6 H).
13C NMR (101 MHz, CDCl3): δ = 160.63, 138.46, 133.38, 131.44, 128.70, 128.29, 126.19, 122.61, 118.09, 115.03, 113.88, 94.80, 61.97, 55.23, 53.68 (d, J J60.63 Hz), 34.99, 29.39, 27.40, 16.52, 16.45.
31P NMR (162 MHz, CDCl3): δ = 26.93.
HRMS (ESI): m/z [M + H]+ calcd for C30H31NO6P: 532.1884; found: 532.1882.
Diethyl(1-(2-methyl-3-phenylcycloprop-2-enyl)-2-(1,3-dioxoisoindolin-2-yl)ethyl)phosphonate (3k)
Yield: 0.011 g (11%); pale-yellow oil.
1H NMR (400 MHz, CDCl3): δ = 7.85–7.87 (m, 2 H), 7.72–7.74 (m, 2 H), 7.58–7.60 (m, 1 H), 7.52–7.54 (m, 1 H), 7.36 (d, J J 7.3 Hz, 1 H), 7.05–7.16 (m, 2 H), 4.98–5.20 (m, 1 H), 4.05–4.15 (m, 4 H), 2.05 (s, 3 H), 1.70 (dd, J , 1.70 (dd, Hz, 3 H), 1.24–1.29 (m, 6 H).
13C NMR (101 MHz, CDCl3): δ = 167.37, 134.11, 133.43, 131.67, 129.14, 128.25, 128.15, 123.49, 122.63, 108.45, 108.05 61.41, 47.60 (d, J J47.60 Hz), 29.69, 21.03, 16.58, 15.16, 14.19.
31P NMR (162 MHz, CDCl3): δ = 27.73.
HRMS (ESI): m/z [M + H]+ calcd for C24H26NO5P: 440.1621; found: 440.1613.
Dimethyl(1-(phenylcycloprop-2-enyl)-2-(1,3-dioxoisoindolin-2-yl)ethyl)phosphonate (3l)
Yield: 0.066 g (55%); pale-yellow oil.
1H NMR (400 MHz, CDCl3): δ = 7.54–7.65 (m, 4 H), 7.48 (d, J = 6.7 Hz, 2 H), 7.17–7.26 (m, 3 H), 7.03 (d, J = 4.2 Hz, 1 H), 5.16–5.25 (m, 1 H), 3.77 (dd, J = 10.6, 5.5 Hz, 6 H), 1.72 (d, J = 7.2 Hz, 3 H).
13C NMR (101 MHz, CDCl3): δ = 168.34, 133.58, 131.46, 129.76, 129.69, 128.38, 125.26, 122.73, 115.73, 97.37, 52.84, 52.70, 47.40 (d, J = 37.1 Hz), 16.07.
31P NMR (162 MHz, CDCl3): δ = 29.30.
HRMS (ESI): m/z [M + H]+ calcd for C21H20NO5P: 398.1113; found: 398.1148.
Dimethyl(1-(phenyl-cycloprop-2-enyl)-2-(1,3-dioxoisoindolin-2-yl)-2-phenyl-ethyl)phosphonate (3m)
Yield: 0.093 g (66%); pale-yellow oil.
1H NMR (400 MHz, CDCl3): δ = 7.45–7.51 (m, 5 H), 7.13–7.24 (m, 7 H), 7.08 (dd, J = 12.8, 5.7 Hz, 2 H), 5.39 (ddd, J = 12.4, 4.3, 2.6 Hz, 1 H), 3.79–3.84(m, 6 H), 3.61–3.74 (m, 2 H), 3.46–3.50 (m, 1 H).
13C NMR (101 MHz, CDCl3): δ = 138.16, 133.49, 129.76, 129.73, 129.63, 128.70, 128.42, 128.35, 126.29, 125.10, 123.48, 122.66, 115.33, 97.46, 53.44 (d, J = 37.0 Hz), 34.94.
31P NMR (162 MHz, CDCl3): δ = 29.11.
HRMS (ESI): m/z [M + H]+ calcd for C27H24NO5P: 474.1426; found: 474.1468.
Diisopropyl(1-(phenylcycloprop-2-enyl)-2-(1,3-dioxoisoindolin-2-yl)-2-phenylethyl)phosphonate (3n)
Yield: 0.094 g (60%); pale-yellow oil.
1H NMR (400 MHz, CDCl3): δ = 7.70 (dd, J = 26.3, 19.1 Hz, 6 H), 7.37–7.51 (m, 3 H), 7.21 (d, J = 4.3 Hz, 1 H), 7.00–7.09 (m, 4 H), 5.44 (d, J = 8.9 Hz, 1 H), 4.52 (ddd, J = 13.4, 11.0, 6.2 Hz, 2 H), 2.74–3.25(m, 2 H), 2.55 (dd, J = 14.0, 4.2 Hz, 1 H), 1.19 (d, J = 6.2 Hz, 6 H), 1.03 (dd, J = 16.5, 6.2 Hz, 6 H).
13C NMR (101 MHz, CDCl3): δ = 153.59, 140.51, 137.65, 133.50, 129.97, 128.85, 128.09.127.98, 127.57, 126.44, 126.29, 123.42, 116.36, 100.21, 70.03 (d, J = 7.4 Hz), 52.71 (d, J = 25.3 Hz), 35.70, 29.71, 23.78.
31P NMR (162 MHz, CDCl3): δ = 24.92.
HRMS (ESI): m/z [M + H]+ calcd for C31H32NO5P: 530.2052; found: 530.2091.
Dibutyl(1-(phenylcycloprop-2-enyl)-2-(1,3-dioxoisoindolin-2-yl)ethyl)phosphonate (3o)
Yield: 0.096 g (67%); pale-yellow oil.
1H NMR (400 MHz, CDCl3): δ = 7.57–7.62 (m, 2 H), 7.52–7.56 (m, 2 H), 7.45 (d, J = 6.8 Hz, 2 H), 7.14–7.23 (m, 3 H), 6.98 (d, J = 4.1 Hz, 1 H), 5.21 (dt, J = 6.9, 5.1 Hz, 1 H), 3.98–4.05 (m, 4 H), 1.70 (d, J = 7.2 Hz, 3 H), 1.56–1.64 (m, 4 H), 1.36 (dd, J = 7.3, 4.6 Hz, 4 H), 0.87–0.92 (m, 6 H).
13C NMR (101 MHz, CDCl3): δ = 168.30, 133.51, 131.50, 129.76, 129.52, 128.23, 125.49, 122.67, 116.09, 97.56, 65.58, 47.49 (d, J = 36.9 Hz), 32.70, 32.41, 18.71, 16.17, 13.58.
31P NMR (162 MHz, CDCl3): δ = 26.73.
HRMS (ESI): m/z [M + H]+ calcd for C27H32NO5P: 482.2052; found: 482.2094.
Dibutyl(1-(phenylcycloprop-2-enyl)-2-(1,3-dioxoisoindolin-2-yl)-2-phenylethyl)phosphonate (3p)
Yield: 0.099 g (60%); pale-yellow oil.
1H NMR (400 MHz, CDCl3): δ = 7.45 (d, J = 8.2 Hz, 5 H), 7.11–7.21 (m, 7 H), 7.05 (d, J = 4.0 Hz, 2 H), 5.36–5.46 (m, 1 H), 4.07 (tt, J = 13.4, 6.7 Hz, 4 H), 3.80 (ddd, J = 31.7, 18.3, 9.8 Hz, 2 H), 3.50 (dd, J = 14.3, 4.1 Hz, 1 H), 1.61–1.68 (m, 4 H), 1.39 (dd, J = 11.2, 4.0 Hz, 4 H), 0.91 (td, J = 7.3, 1.4 Hz, 6 H).
13C NMR (101 MHz, CDCl3): δ = 138.38, 133.43, 129.75, 129.59, 128.68, 128.33, 128.30, 126.23, 125.36, 123.68, 122.62, 115.62, 97.59, 65.74, 53.60 (d, J = 37.3 Hz), 35.02, 32.66, 29.68, 18.78, 13.66.
31P NMR (162 MHz, CDCl3): δ = 26.53.
HRMS (ESI): m/z [M + H]+ calcd for C33H36NO5P: 558.2365; found: 558.2412.
(Z)-Diethyl(2-(1,3-dioxoisoindolin-2-yl)prop-1-en-1-yl)phosphonate (4a)
Yield: 0.014 g (15%); white solid; mp 140–143 °C.
1H NMR (400 MHz, CDCl3): δ = 7.87–7.94 (m, 2 H, Ph), 7.69–7.80 (m, 2 H, Ph), 5.96 (dd, J = 10.4, 1.2 Hz, 1 H, CH), 3.98–4.13 (m, 4 H, 2 °CH2), 2.25 (s, 3 H, CH3), 1.28 (t, J = 7.1 Hz, 6 H, 2CH3).
13C NMR (101 MHz, CDCl3): δ = 166.63 (C=O), 146.83 (CN), 134.11, 132.28, 123.71 (Ph), 118.32 (d, J = 186.2 Hz, CP), 62.08 (d, J = 5.3 Hz, OCH2), 24.56 (d, J = 17 Hz, CH3), 16.27 (d, J = 6.6 Hz, CH3).
31P NMR (162 MHz, CDCl3): δ = 11.34 (s).
HRMS (ESI): m/z [M + Na]+ calcd for C15H18NO5PNa: 346.0815; found: 346.0815.
Supporting Information
- Supporting information for this article is available online at https://doi.org/10.1055/s-0036-1590969.
- Supporting Information
-
References
- 1a Rubin M. Rubina M. Gevorgyan V. Chem. Rev. 2007; 107: 3117
- 1b Rubin M. Rubina M. Gevorgyan V. Synthesis 2006; 1221
- 1c Fox JM. Yan N. Curr. Org. Chem. 2005; 9: 719
- 1d Nakamura M. Isobe H. Nakamura E. Chem. Rev. 2003; 103: 1295
- 1e Song CL. Wang JW. Xu ZH. Acta Chim. Sin. 2015; 73: 1114
- 1f Prosser AR. Banning JE. Rubina M. Rubin M. Org. Lett. 2010; 12: 3968
- 1g Banning JE. Prosser AR. Rubin M. Org. Lett. 2010; 12: 1488
- 1h Alnasleh BK. Sherrill WM. Rubina M. Banning J. Rubin M. J. Am. Chem. Soc. 2009; 131: 6906
- 1i Sherrill WM. Rubin M. J. Am. Chem. Soc. 2008; 130: 13804
- 1j Alnasleh BK. Sherrill WM. Rubin M. Org. Lett. 2008; 10: 3231
- 1k Xie XC. Yang Z. Fox JM. J. Org. Chem. 2010; 75: 3847
- 1l Tarwade V. Liu XZ. Yan N. Fox JM. J. Am. Chem. Soc. 2009; 131: 5382
- 1m Yan N. Liu XZ. Fox JM. J. Org. Chem. 2008; 73: 563
- 1n Fisher LA. Fox JM. J. Org. Chem. 2008; 73: 8474
- 1o Simaan M. Delaye PO. Shi M. Angew. Chem. Int. Ed. 2015; 54: 12345
- 1p Song CL. Wang JW. Xu ZH. Org. Biomol. Chem. 2014; 12: 5802
- 1q Wallbaum J. Jones PG. Werz DB. J. Org. Chem. 2015; 80: 3730
- 1r Tian ZX. Lis L. Kass SR. J. Org. Chem. 2013; 78: 12650
- 2a Simaan S. Masarwa A. Zohar E. Stanger A. Bertus P. Marek I. Chem. Eur. J. 2009; 15: 8449
- 2b Hoveyda AH. Lombardi PJ. O’Brien RV. Zhugralin AR. J. Am. Chem. Soc. 2009; 131: 8378
- 2c Marek I. Simaan S. Masarwa A. Angew. Chem. Int. Ed. 2007; 46: 7364
- 2d Sherill WM. Rubin M. J. Am. Chem. Soc. 2008; 130: 13804
- 2e Tarwade V. Liu X. Yan N. Fox JM. J. Am. Chem. Soc. 2009; 131: 5382
- 2f Briones JF. Davies HM. L. J. Am. Chem. Soc. 2012; 134: 11916
- 2g Zhu ZB. Wei Y. Shi M. Chem. Soc. Rev. 2011; 40: 5534
- 2h Lindsay VN. G. Fiest D. Gritsch PJ. Azzi S. Charette AB. J. Am. Chem. Soc. 2013; 135: 1463
- 3a Perez PJ. Brookhart M. Templeton JL. Organometallics 1993; 12: 261
- 3b Cho DJ. Jeon SJ. Kim HS. Cho CS. Shim SC. Kim TJ. Tetrahedron: Asymmetry 1999; 10: 3833
- 3c Doyle MP. Hu WH. Tetrahedron Lett. 2000; 41: 6265
- 3d Doyle MP. Hu WH. Synlett 2001; 9: 1364
- 3e Swenson AK. Higgins KE. Brewer MG. Org. Biomol. Chem. 2012; 10: 7483
- 3f Martin C. Sierra M. Alvarez T. Belderrain TR. Perez PJ. Dalton Trans. 2012; 5319
- 3g Thomas TJ. Merritt BA. Lemma BE. Org. Biomol. Chem. 2016; 14: 1742
- 4 Uehara M. Suematsu H. Yasutomi Y. Katsuki T. J. Am. Chem. Soc. 2011; 133: 170
- 5a Cui X. Xu X. Lu HJ. Zhu SF. Woitas L. Zhang XP. J. Am. Chem. Soc. 2011; 133: 3304
- 5b Cui X. Xu X. Woitas L. Kim MM. Zhang XP. J. Am. Chem. Soc. 2012; 134: 19981
- 6a Weatherhead-Kloster RA. Corey EJ. Org. Lett. 2006; 8: 171
- 6b Chuprakov S. Gevorgyan V. Org. Lett. 2007; 9: 4463
- 6c Briones JF. Hansen J. Hardcastle KI. Autschbach J. Davies HM. L. J. Am. Chem. Soc. 2010; 132: 17211
- 6d Panne P. Fox JM. J. Am. Chem. Soc. 2007; 129: 22
- 6e Morandi B. Carreira EM. Angew. Chem. Int. Ed. 2010; 49: 4294
- 6f Goto T. Takeda K. Shimada N. Angew. Chem. Int. Ed. 2011; 50: 6803
- 6g Briones JF. Davies HM. L. Tetrahedron 2011; 67: 4313
- 6h Xu XF. Deng YM. Yim DN. Zavalij PY. Doyle MP. Chem. Sci. 2015; 6: 2196
- 6i Edwards A. Rubin M. Tetrahedron 2015; 71: 3237
- 7a Wu CG. Liu ZX. Zhang ZK. Ye F. Deng GS. Zhang Y. Wang JB. Adv. Synth. Catal. 2016; 358: 2480
- 7b Zhou YJ. Ye F. Zhou Q. Zhang Y. Wang JB. Org. Lett. 2016; 18: 2024
- 8a Protopopova MN. Doyle MP. Müller P. Ene D. J. Am. Chem. Soc. 1992; 114: 2755
- 8b Doyle MP. Protopopova M. Müller P. Ene D. Shapiro EA. J. Am. Chem. Soc. 1994; 116: 8492
- 8c Doyle MP. Ene D. Peterson CS. Lynch V. Angew. Chem. Int. Ed. 1999; 38: 700
- 9 Lou Y. Horikawa M. Kloster RA. Hawryluk NA. Corey EJ. J. Am. Chem. Soc. 2004; 126: 8916
- 10 Davies HM. L. Lee GH. Org. Lett. 2004; 6: 1233
- 11 Briones JF. Davies HM. L. Org. Lett. 2011; 13: 3984
- 12a Zhu SF. Chen WQ. Zhang QQ. Mao HX. Zhou QL. Synlett 2011; 7: 919
- 12b Zhou CY. Wang JC. Wei JH. Xu ZJ. Guo Z. Low KH. Che CM. Angew. Chem. Int. Ed. 2012; 51: 11376
- 12c Lindsay VN. G. Fiset D. Gritsch PJ. Azzi S. Charette AB. J. Am. Chem. Soc. 2013; 135: 1463
- 12d Wang J. Boyarshikh V. Rainier JD. Org. Lett. 2011; 13: 700
- 12e Davies HM. L. Lee GH. Org. Lett. 2004; 6: 2117
- 12f Zhang H. Wen XJ. Gan LH. Peng YG. Org. Lett. 2012; 14: 2126
- 12g Hashimoto T. Maruoka K. J. Am. Chem. Soc. 2007; 129: 10054
- 12h Marmor RS. Seyferth D. J. Org. Chem. 1971; 36: 128
- 13 Lindsay VN. G. Fiset D. Gritsch PJ. Azzi S. Charette AB. J. Am. Chem. Soc. 2013; 135: 1463
- 14a Pyun HJ. Chaudhary K. Somoza JZ. Sheng XC. Kim UC. Tetrahedron Lett. 2009; 50: 3833
- 14b Sheng XC. Pyun H.-J. Chaudhary K. Wang J. Doerffler E. Fleury M. McMurtrie D. Chen X. Delaney IW. E. Kim CU. Bioorg. Med. Chem. Lett. 2009; 19: 3453
- 14c Clarke MO. Chen X. Cho A. Delaney IW. E. Doerffler E. Fardis M. Ji M. Mertzman M. Pakdaman R. Pyun H.-J. Rowe T. Yang CY. Sheng XC. Kim CU. Bioorg. Med. Chem. Lett. 2011; 21: 3568
- 14d Sheng XC. Casarez A. Cai R. Clarke MO. Chen X. Cho A. Delaney IW. E. Doerffler E. Ji M. Mertzman M. Pakdaman R. Pyun H.-J. Rowe T. Wu Q. Xu J. Kim CU. Bioorg. Med. Chem. Lett. 2012; 22: 1394
- 14e Gulevich AV. Helan V. Wink DJ. Gevorgyan V. Org. Lett. 2013; 15: 956
- 15a Moore JD. Sprott KT. Wrobleski AD. Hanson PR. Org. Lett. 2002; 4: 2357
- 15b Moore JD. Hanson PR. Tetrahedron: Asymmetry 2003; 14: 873
- 15c Chemagin AV. Yashin NV. Grishin YK. Kuznetsova TS. Zefirov NS. Synthesis 2010; 259
- 15d Chemagin AV. Yashin NV. Grishin YK. Kuznetsova TS. Zefirov NS. Synthesis 2010; 3379
- 16 Cai Y. Lu YC. Yu CB. Lyu HR. Miao ZW. Org. Biomol. Chem. 2013; 11: 5491
- 17 Cai Y. Ge HH. Sun WZ. Miao ZW. Synthesis 2015; 47: 1669
- 18a Kong SS. Fan WD. Wu GP. Miao ZW. Angew. Chem. Int. Ed. 2012; 51: 8864
- 18b Fang ZJ. Yang HH. Miao ZW. Chen RY. Helv. Chim. Acta 2011; 94: 1586
- 18c Wang YD. Wang YY. Yu JP. Miao ZW. Chen RY. Chem. Eur. J. 2009; 15: 9290
- 18d Wang YD. Wang F. Wang YY. Miao ZW. Chen RY. Adv. Synth. Catal. 2008; 350: 2339
- 18e Cai Y. Lyu HR. Yu CB. Miao ZW. Adv. Synth. Catal. 2014; 356: 596
- 18f Cai Y. Ge HH. Yu CB. Sun WZ. Zhan JC. Miao ZW. RSC Adv. 2014; 4: 21492
- 18g Ge HH. Liu S. Cai Y. Sun YC. Miao ZW. Synthesis 2016; 48: 448
- 19 See the Supporting Information.
-
References
- 1a Rubin M. Rubina M. Gevorgyan V. Chem. Rev. 2007; 107: 3117
- 1b Rubin M. Rubina M. Gevorgyan V. Synthesis 2006; 1221
- 1c Fox JM. Yan N. Curr. Org. Chem. 2005; 9: 719
- 1d Nakamura M. Isobe H. Nakamura E. Chem. Rev. 2003; 103: 1295
- 1e Song CL. Wang JW. Xu ZH. Acta Chim. Sin. 2015; 73: 1114
- 1f Prosser AR. Banning JE. Rubina M. Rubin M. Org. Lett. 2010; 12: 3968
- 1g Banning JE. Prosser AR. Rubin M. Org. Lett. 2010; 12: 1488
- 1h Alnasleh BK. Sherrill WM. Rubina M. Banning J. Rubin M. J. Am. Chem. Soc. 2009; 131: 6906
- 1i Sherrill WM. Rubin M. J. Am. Chem. Soc. 2008; 130: 13804
- 1j Alnasleh BK. Sherrill WM. Rubin M. Org. Lett. 2008; 10: 3231
- 1k Xie XC. Yang Z. Fox JM. J. Org. Chem. 2010; 75: 3847
- 1l Tarwade V. Liu XZ. Yan N. Fox JM. J. Am. Chem. Soc. 2009; 131: 5382
- 1m Yan N. Liu XZ. Fox JM. J. Org. Chem. 2008; 73: 563
- 1n Fisher LA. Fox JM. J. Org. Chem. 2008; 73: 8474
- 1o Simaan M. Delaye PO. Shi M. Angew. Chem. Int. Ed. 2015; 54: 12345
- 1p Song CL. Wang JW. Xu ZH. Org. Biomol. Chem. 2014; 12: 5802
- 1q Wallbaum J. Jones PG. Werz DB. J. Org. Chem. 2015; 80: 3730
- 1r Tian ZX. Lis L. Kass SR. J. Org. Chem. 2013; 78: 12650
- 2a Simaan S. Masarwa A. Zohar E. Stanger A. Bertus P. Marek I. Chem. Eur. J. 2009; 15: 8449
- 2b Hoveyda AH. Lombardi PJ. O’Brien RV. Zhugralin AR. J. Am. Chem. Soc. 2009; 131: 8378
- 2c Marek I. Simaan S. Masarwa A. Angew. Chem. Int. Ed. 2007; 46: 7364
- 2d Sherill WM. Rubin M. J. Am. Chem. Soc. 2008; 130: 13804
- 2e Tarwade V. Liu X. Yan N. Fox JM. J. Am. Chem. Soc. 2009; 131: 5382
- 2f Briones JF. Davies HM. L. J. Am. Chem. Soc. 2012; 134: 11916
- 2g Zhu ZB. Wei Y. Shi M. Chem. Soc. Rev. 2011; 40: 5534
- 2h Lindsay VN. G. Fiest D. Gritsch PJ. Azzi S. Charette AB. J. Am. Chem. Soc. 2013; 135: 1463
- 3a Perez PJ. Brookhart M. Templeton JL. Organometallics 1993; 12: 261
- 3b Cho DJ. Jeon SJ. Kim HS. Cho CS. Shim SC. Kim TJ. Tetrahedron: Asymmetry 1999; 10: 3833
- 3c Doyle MP. Hu WH. Tetrahedron Lett. 2000; 41: 6265
- 3d Doyle MP. Hu WH. Synlett 2001; 9: 1364
- 3e Swenson AK. Higgins KE. Brewer MG. Org. Biomol. Chem. 2012; 10: 7483
- 3f Martin C. Sierra M. Alvarez T. Belderrain TR. Perez PJ. Dalton Trans. 2012; 5319
- 3g Thomas TJ. Merritt BA. Lemma BE. Org. Biomol. Chem. 2016; 14: 1742
- 4 Uehara M. Suematsu H. Yasutomi Y. Katsuki T. J. Am. Chem. Soc. 2011; 133: 170
- 5a Cui X. Xu X. Lu HJ. Zhu SF. Woitas L. Zhang XP. J. Am. Chem. Soc. 2011; 133: 3304
- 5b Cui X. Xu X. Woitas L. Kim MM. Zhang XP. J. Am. Chem. Soc. 2012; 134: 19981
- 6a Weatherhead-Kloster RA. Corey EJ. Org. Lett. 2006; 8: 171
- 6b Chuprakov S. Gevorgyan V. Org. Lett. 2007; 9: 4463
- 6c Briones JF. Hansen J. Hardcastle KI. Autschbach J. Davies HM. L. J. Am. Chem. Soc. 2010; 132: 17211
- 6d Panne P. Fox JM. J. Am. Chem. Soc. 2007; 129: 22
- 6e Morandi B. Carreira EM. Angew. Chem. Int. Ed. 2010; 49: 4294
- 6f Goto T. Takeda K. Shimada N. Angew. Chem. Int. Ed. 2011; 50: 6803
- 6g Briones JF. Davies HM. L. Tetrahedron 2011; 67: 4313
- 6h Xu XF. Deng YM. Yim DN. Zavalij PY. Doyle MP. Chem. Sci. 2015; 6: 2196
- 6i Edwards A. Rubin M. Tetrahedron 2015; 71: 3237
- 7a Wu CG. Liu ZX. Zhang ZK. Ye F. Deng GS. Zhang Y. Wang JB. Adv. Synth. Catal. 2016; 358: 2480
- 7b Zhou YJ. Ye F. Zhou Q. Zhang Y. Wang JB. Org. Lett. 2016; 18: 2024
- 8a Protopopova MN. Doyle MP. Müller P. Ene D. J. Am. Chem. Soc. 1992; 114: 2755
- 8b Doyle MP. Protopopova M. Müller P. Ene D. Shapiro EA. J. Am. Chem. Soc. 1994; 116: 8492
- 8c Doyle MP. Ene D. Peterson CS. Lynch V. Angew. Chem. Int. Ed. 1999; 38: 700
- 9 Lou Y. Horikawa M. Kloster RA. Hawryluk NA. Corey EJ. J. Am. Chem. Soc. 2004; 126: 8916
- 10 Davies HM. L. Lee GH. Org. Lett. 2004; 6: 1233
- 11 Briones JF. Davies HM. L. Org. Lett. 2011; 13: 3984
- 12a Zhu SF. Chen WQ. Zhang QQ. Mao HX. Zhou QL. Synlett 2011; 7: 919
- 12b Zhou CY. Wang JC. Wei JH. Xu ZJ. Guo Z. Low KH. Che CM. Angew. Chem. Int. Ed. 2012; 51: 11376
- 12c Lindsay VN. G. Fiset D. Gritsch PJ. Azzi S. Charette AB. J. Am. Chem. Soc. 2013; 135: 1463
- 12d Wang J. Boyarshikh V. Rainier JD. Org. Lett. 2011; 13: 700
- 12e Davies HM. L. Lee GH. Org. Lett. 2004; 6: 2117
- 12f Zhang H. Wen XJ. Gan LH. Peng YG. Org. Lett. 2012; 14: 2126
- 12g Hashimoto T. Maruoka K. J. Am. Chem. Soc. 2007; 129: 10054
- 12h Marmor RS. Seyferth D. J. Org. Chem. 1971; 36: 128
- 13 Lindsay VN. G. Fiset D. Gritsch PJ. Azzi S. Charette AB. J. Am. Chem. Soc. 2013; 135: 1463
- 14a Pyun HJ. Chaudhary K. Somoza JZ. Sheng XC. Kim UC. Tetrahedron Lett. 2009; 50: 3833
- 14b Sheng XC. Pyun H.-J. Chaudhary K. Wang J. Doerffler E. Fleury M. McMurtrie D. Chen X. Delaney IW. E. Kim CU. Bioorg. Med. Chem. Lett. 2009; 19: 3453
- 14c Clarke MO. Chen X. Cho A. Delaney IW. E. Doerffler E. Fardis M. Ji M. Mertzman M. Pakdaman R. Pyun H.-J. Rowe T. Yang CY. Sheng XC. Kim CU. Bioorg. Med. Chem. Lett. 2011; 21: 3568
- 14d Sheng XC. Casarez A. Cai R. Clarke MO. Chen X. Cho A. Delaney IW. E. Doerffler E. Ji M. Mertzman M. Pakdaman R. Pyun H.-J. Rowe T. Wu Q. Xu J. Kim CU. Bioorg. Med. Chem. Lett. 2012; 22: 1394
- 14e Gulevich AV. Helan V. Wink DJ. Gevorgyan V. Org. Lett. 2013; 15: 956
- 15a Moore JD. Sprott KT. Wrobleski AD. Hanson PR. Org. Lett. 2002; 4: 2357
- 15b Moore JD. Hanson PR. Tetrahedron: Asymmetry 2003; 14: 873
- 15c Chemagin AV. Yashin NV. Grishin YK. Kuznetsova TS. Zefirov NS. Synthesis 2010; 259
- 15d Chemagin AV. Yashin NV. Grishin YK. Kuznetsova TS. Zefirov NS. Synthesis 2010; 3379
- 16 Cai Y. Lu YC. Yu CB. Lyu HR. Miao ZW. Org. Biomol. Chem. 2013; 11: 5491
- 17 Cai Y. Ge HH. Sun WZ. Miao ZW. Synthesis 2015; 47: 1669
- 18a Kong SS. Fan WD. Wu GP. Miao ZW. Angew. Chem. Int. Ed. 2012; 51: 8864
- 18b Fang ZJ. Yang HH. Miao ZW. Chen RY. Helv. Chim. Acta 2011; 94: 1586
- 18c Wang YD. Wang YY. Yu JP. Miao ZW. Chen RY. Chem. Eur. J. 2009; 15: 9290
- 18d Wang YD. Wang F. Wang YY. Miao ZW. Chen RY. Adv. Synth. Catal. 2008; 350: 2339
- 18e Cai Y. Lyu HR. Yu CB. Miao ZW. Adv. Synth. Catal. 2014; 356: 596
- 18f Cai Y. Ge HH. Yu CB. Sun WZ. Zhan JC. Miao ZW. RSC Adv. 2014; 4: 21492
- 18g Ge HH. Liu S. Cai Y. Sun YC. Miao ZW. Synthesis 2016; 48: 448
- 19 See the Supporting Information.




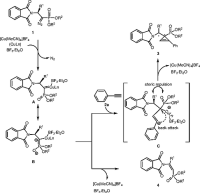
